NASA Medical Research Studies
You didn’t come this far to stop
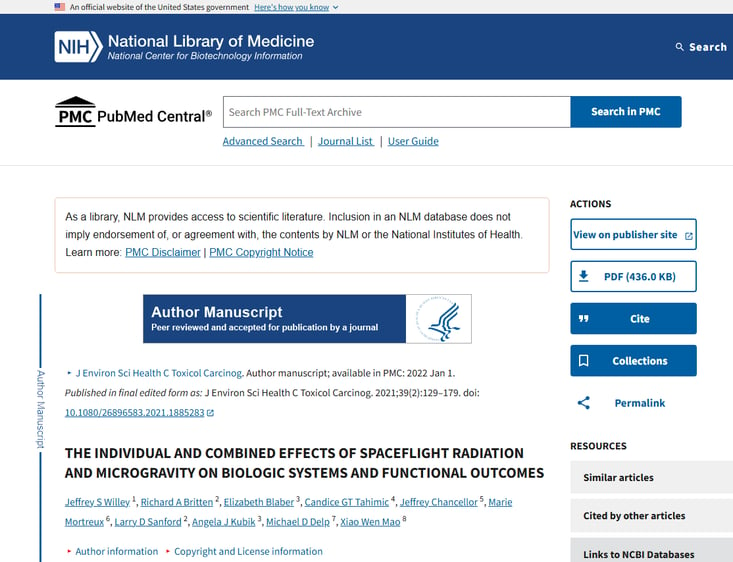
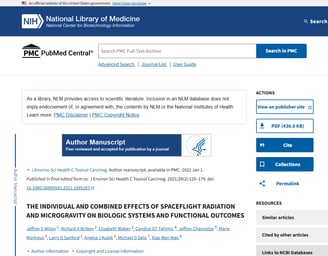
OVERVIEW
Loss of gravitational loading on the body and across tissues results in multisystem injury6.
Among musculoskeletal tissues that exhibit a dynamic response to loading14–16, exposure to microgravity results in: muscle atrophy17,18, loss of bone mineral density primarily at load-bearing skeletal elements (e.g., pelvis, femur, vertebrae)19–21, and increased risk of herniation of the intervertebral disks due to increased fluid volume22,23.
These negative effects also could be compounded by spinal muscle atrophy18 and affected spinal ligaments, ultimately leading to pain23–25.
Cardiovascular responses to microgravity include redistribution of blood cranially, increasing arterial pressure at the head and lowering pressure at the distal leg elements26; though the response may be transient.
This altered pressure gradient may contribute to spaceflight-associated neuro-ocular syndrome3 in which optic disk edema, optic globe flattening, thickening or folding of the choroid layer, ischemic regions of the retina, and hyperopic visual disturbance may occur27.
SKELETAL RESPONSE
Bone Loss in Microgravity and Reduced Weight-Bearing:
Spaceflight environmental conditions, particularly microgravity and low-dose radiation, represent a risk to astronaut bone health.
In the absence of mitigation strategies, spaceflight can lead to decrements in bone mass and strength85.
Crew members returning from a 4–6 month mission on the ISS had reduced areal bone mineral density (aBMD) of the hip and spine.
The rate of bone loss was found to be site-specific, with a 1.4–1.5% and 0.9% decrease in aBMD of the hip and spine respectively per month in the ISS.
In the hip, the rate of loss was higher in cancellous bone (2.2–2.7%/month) compared to cortical bone (0.4–0.5%/month)86.
In contrast, trabecular vBMD and mass decreased by 14.4–16.5 % throughout the mission duration87.
Bone strength in the proximal femur also was reduced as determined by finite element modeling for stance and fall loading21.
These decrements in skeletal structure and strength may increase the risk of fractures, which in turn can be catastrophic to future long duration spaceflight missions where there will be operational constraints on medical care and equipment.
Space Radiation-induced Bone Damage:
Bone loss also has been examined in animal models for space radiation exposure.
Historically, bone was considered radiation resistant, and thus the dose threshold for damage was assumed to be high91,92.
However, studies performed in the early portion of the 2000’s identified late bone loss in mice after 2 Gy exposures to multiple qualities of radiation (e.g., protons, iron ions, carbon ions, and photons), with the consideration that 2 Gy represented an appropriate comparative dose between clinical fractions and spaceflight exposures93.
Later studies typically made use of a lower reference dose of ionizing radiation (e.g. 1–2 Gy 137Cs)73,81,94, a single representative species of GCR35,84,95,96 or sequential exposure to two ion species72,97.
These spaceflight relevant studies identified that while osteoblast activity is lower after exposure (which historically was assumed to be the mediator of late bone damage after high dose exposure), osteoclast activity was increased as an early response98,99, leading to acute bone loss78,98.
The dose threshold for bone loss after simulated spaceflight radiation was determined to be low, with mixed fragment doses <0.5 Sv (protons and helium ions) causing late bone (and muscle) damage100, and individual doses of 0.5 Gy 56Fe and protons causing acute bone loss in mice101.
These investigations have thus confirmed that bone is a radiation sensitive tissue, with the outcome being loss of architecture and density.
However, one study has reported that radiation can have positive effects on bone microarchitecture74.
Skeletally mature (16 week old) female mice exposed to 0.5 Gy 56Fe exhibited increased cancellous % bone volume (BV/TV), trabecular thickness (Tb.Th.) and trabecular number (Tb.N.) in the distal femur at 21 days post-irradiation.
These results highlight that while radiation is generally considered disadvantageous, many questions remain regarding how low dose radiation could affect bone, including questions regarding bone material and mechanical properties that occur with alterations in architectural properties.
These questions should be addressed in the context of complex, mixed beams of energetic particles to improve the model spaceflight-relevant radiation exposures.
Recently, the NSRL has developed a GCR simulation involving a more complex combination of multiple ion species that approximates the anticipated exposure of crew based on current spacecraft design102.
Impact of Combined Exposure of Space Radiation and Simulated Microgravity on Bone Structure and Function:
Published studies on the combined effects of microgravity and radiation on skeletal integrity differ in experiment designs, making comparison and validation of results across studies quite challenging.
Despite the limited number of reports and heterogeneity in experiment designs, two major conclusions can be drawn from collective findings.
Firstly, findings from animal models predict that bone loss due to microgravity and ionizing radiation exposure can be additive under certain conditions.
Secondly, skeletal parameters may have differential sensitivities to combined spaceflight factors.
In some studies that have used representative high linear energy transfer (LET) species in combination with HLU, additive effects were seen in a subset of skeletal structural parameters but not in biomechanical properties.
For example, 16-week old, male C57BL/6J mice that underwent 14 days of HLU displayed decreased cancellous and cortical BV/TV in tibia versus normally loaded controls while similarly aged cohorts exposed to 50 cGy proton or 50 cGy proton + 10 cGy 16O did not show such decrements.
Yet when HLU was combined with 50 cGy proton + 10 cGy 16O exposure, further decrements in BV/TV were observed.
Biomechanical properties such as maximal force, measured by three-point bending, was negatively impacted by HLU but not by radiation.
However, no further deficits were observed when HLU and radiation were combined72.
These findings are generally consistent with another report which made use of similarly aged female mice35 exposed to 100 cGy of protons in combination with four weeks of HLU.
Combined microgravity and radiation also can lead to skeletal impairments at the cellular level.
Skeletal homeostasis is achieved through the balance in the activity of bone-forming osteoblasts and bone-resorbing osteoclasts.
Exposure to 0.5 Gy 56Fe was found to exacerbate HLU-induced deficits in alkaline phosphatase activity of marrow-derived ex vivo osteoblast cultures, suggesting that microgravity in combination with radiation exposure can impair osteoblastogenesis96.
Additionally, two weeks of HLU combined with 1 Gy 56Fe delivered on Day 3 led to enhanced bone loss versus exposure to each single hazard.
This study also identified potential mechanisms for bone loss in the hind limb from each challenge, related to endothelial dependent vasodilation of the feed arteries of the gastrocnemius83.
Specifically, it was identified that altered vasodilation associated with bone loss was mediated by altered nitric oxide (NO) synthase signaling, although occurring in divergent manners between hazards: with HLU, alterations occurred to reduced production and concentration of NO, and radiation-induced alterations were due to increased NO quenching.
Long duration missions will involve extended periods of exposure to low doses of space radiation.
Most of the available data on the effects of radiation on the skeleton were generated from acute exposures.
Published results from fractionated or sustained exposure models remain a rarity.
One group has examined the effects of 0.5 Gy 28Si delivered as an acute dose or three fractionated doses of 0.17 Gy in combination with PWB at one sixth body weight95.
The PWB-induced decrements in both endocortical and periosteal % mineralizing surfaces of cortical bone sites appeared to be worsened by 28Si although standard deviations were wide, therefore necessitating additional confirmatory studies95.
In general, neither acute nor fractionated exposure exacerbated the effects of partial weight-bearing on femoral BV/TV and femoral neck biomechanical properties such as load to failure and stiffness.
Others who have simultaneously applied continuous exposure to low dose rate photons (8.5cGy 137Cs) exhibited no radiation response, only bone loss due to HLU over the course of a 20 day study81.
The long-term consequences of combined microgravity and radiation on skeletal health is unclear given that most investigations have focused on immediate time points (e.g. assessment after unloading).
However, there is evidence that combined exposure to HLU and HZE (0.5 Gy) can negatively impact skeletal recovery after a period of unloading.
In one such study84, animals that received an acute dose of 0.5 Gy 56Fe within a 14-day period of HLU displayed persistent deficits in vertebral trabecular morphology (structural model index, SMI) after a 28-day period of re-ambulation.
However, cancellous BV/TV of vertebra of these animals were comparable to untreated controls after the same time period.
Humans living in space experience circadian misalignment103.
The interaction of the circadian clock with microgravity and space radiation has not been well examined with alterations in bone as an outcome.
One investigation exposed rats to an acute, high dose of radiation (4 Gy 137Cs), with ultradian cycles (45 min light/45 min dark), HLU or all factors combined.
Ultradian cycles were sufficient to induce decrements in bone biomechanical properties but did not lead to bone loss.
In addition, ultraradian rhythms did not worsen the negative effects of HLU and radiation on bone structure and strength.
Countermeasures in place to prevent osteopenia during ISS missions include load-bearing exercises.
Strides also have been made in identifying other promising candidate countermeasures for mitigating spaceflight-induced bone loss.
Antioxidants are often considered as candidate countermeasures to protect against radiation and/or HLU-induced bone loss: as previously noted altered NO concentration (and expanded upon in Section 5; Cardiovascular Response) is associated with radiation and HLU83, and biomarkers for oxidative stress in the marrow in another study has been identified to increase after radiation but not HLU78, highlighting some inconsistencies in the literature.
In a rodent model, pre-feeding with an antioxidant-rich dietary supplement (dried plum) prevented bone loss and decrements in bone strength resulting from HLU, ionizing radiation and in combination94.
These results suggest some shared mechanisms underlying microgravity and radiation-induced bone loss.
However, while antioxidants such as dihydrolipoic acid (DHLA) or an antioxidant cocktail have been shown to be less efficacious at reducing bone loss in mice early after 2 Gy gamma ray exposure,97 alpha-lipoic acid78 has been shown to reduce early bone loss after 2 Gy gamma rays.
Collectively, these findings suggest that antioxidants have varying efficacies in preventing the negative effects of spaceflight stressors on bone.
Future studies are needed to better understand the underlying mechanisms for the protective effects of promising antioxidant-based countermeasures for spaceflight.
Additional and Future Considerations:
Although much has been achieved in understanding the effects of spaceflight on bone health, follow-up studies are needed to validate the abovementioned findings using improved radiation exposure paradigms such as the recently developed multi-ion GCR simulation102.
The use of animals that better match the age of mission crew when performing spaceflight simulation studies will facilitate translation of rodent data to humans in space.
In addition, standardization of methodologies and caging designs for conducting HLU and partial weight-bearing studies also are important to allow for comparison and interpretation of findings across studies and research groups.
More mechanistic investigations also are needed to understand the molecular underpinnings of the skeletal response to combined spaceflight factors.
Bioinformatics approaches that allow for the assessment of global changes at the genome, transcriptome and proteome levels are particularly useful in identifying novel pathways that underlie the skeletal response to spaceflight.
To date, omics data from skeletal tissue is rare.
Of the two traditionally mechanosensitive tissues, muscle vastly outnumbers bone or bone cell transcriptomic datasets found on the NASA GeneLab database.
Increasing the availability of bone omics datasets will facilitate improved understanding of skeletal signaling in response to spaceflight.
Initiatives to study bone health in space have predominantly focused on how spaceflight negatively impacts skeletal structure and biomechanical properties with the overarching goal of understanding how they contribute to fracture risk.
While it is important to address remaining knowledge gaps on fracture risk in follow-up studies, investigations by the space research community also need to broaden to reflect our growing understanding of skeletal function.
Beyond providing structural support, the skeleton is an endocrine organ that can crosstalk with other tissues to maintain health and homeostasis.
A number of studies demonstrate the ability of bone to function as an endocrine organ (reviewed in104–106 to regulate a variety of physiological processes including glucose metabolism107–109, appetite suppression110, cognition and behavior111,112.
The organism’s ability to coordinate the function of multiple tissues via bone-derived factors is essential to keep up with the demands of daily living.
For example, bone-derived lipocalin-2 (LCN2) regulates glucose homeostasis via endocrine action on major metabolic organs, and also can cross the blood-brain barrier to control appetite via its binding to the melanocortin receptor (MC4R) in the hypothalamus110.
In addition, LCN2 can exert pro-inflammatory actions on a variety of cell types including vascular cells113.
Osteocalcin (OCN), another bone-derived hormone can modulate cognition and anxiety-like behavior111,112.
Mice heterozygous for an OCN null allele showed deficits in cognition while administration of OCN improved memory and decreased anxiety-like behaviors112.
In addition, OCN has been shown to mediate aspects of the acute stress response.
In the presence of stressors, it is thought that OCN participates in signaling to inhibit the parasympathetic branch of the autonomic nervous system to allow the sympathetic pathway to predominate, in turn promoting flight or fight responses114.
The biological processes regulated by these bone-derived hormones are critical for human health and performance in space missions.
Hence, it is important to begin to address whether combined spaceflight factors can perturb signaling mediated by bone-derived hormones.
More studies also are needed to understand the role of bone crosstalk with other organs in mediating the physiological changes attributed to spaceflight.
3. OCULAR RESPONSE
There has been an increase in the incidence of the ocular problem reported in astronauts during and after space shuttle missions or orbits aboard ISS3.
As previously noted, this syndrome, known as spaceflight associated neuro-ocular syndrome (SANS), is characterized by pathophysiology symptoms including optic disc edema, globe flattening, choroidal and retinal folds, hyperopic refractive error shifts, and nerve fiber layer infarcts (i.e., cotton wool spots)115–117.
In the last decade, over 30% of astronauts flying long-duration ISS missions have presented with one or more of these ocular disturbances118.
Most recently, the NASA twin study found variations in choroidal and total retinal thickness, which was suggestive of retinal edema and choroidal folds in the twin exposed to spaceflight119.
There is concern that degradation of visual function as a result of space flight may compromise both mission goals and long-term quality of life after space travel.
Ocular damage and retinal degeneration can be promoted by many factors including aging, ischemia, fluctuation in oxygen tension, oxidative stress, and increased intraocular pressure120.
Visual disturbances associated with space travel may be due to exposure from altered gravitation changes and ionizing radiation116,120–122.
Although some ocular changes experienced by astronauts have been measured and evaluated117,118, validation was difficult due to the limited subject cohort size and test constraints on ISS.
Furthermore, the underlying mechanisms of these ocular disturbance and factors contributing to the development of damage are currently unclear.
Comprehensive ground-based rodent study models to simulate space condition including low-dose ionizing radiation and microgravity is warranted to determine the impact of the space environment on ocular structure and function.
Microgravity-induced Ocular Damage:
Microgravity induces a cephalic shift in body fluids, an increase in cephalad shifting of body fluids, and alterations in tissue perfusion121,123,124.
Increasingly, evidence suggests that both actual microgravity encountered by astronauts in space, as well as modeled microgravity on Earth, have been shown to induce many deleterious physiological effects including changes in ocular structure and function125,126.
After long-duration spaceflight, morphological changes in the optic nerve and surrounding tissues have been reported127.
One study showed that even in transient microgravity conditions, as produced by parabolic flight, changes in retinal vasculature occur117.
Microgravity may also induce an increase in intraocular pressure (IOP)128.
A more recent study reports that in astronauts, there is an acute increase in IOP upon entering weightlessness, but that it normalizes to ground-based levels after a few days of flight118.
Despite reported observations, cellular mechanisms of microgravity in inducing the unique physiological and pathological ocular responses have not yet been well-understood.
Moreover, head-down tilt (HDT) during bed rest as a ground-based, human analog for microgravity has not confirmed some of SANS findings in astronauts129.
Space Radiation-induced Ocular Damage:
The adverse effects of radiation on the retina130–133 and retinal vasculature132,134 have been reported by multiple investigators who have documented structural, histopathological, and functional alterations in the affected retina after irradiation exposure.
Despite reported studies, most of the animal and clinical investigations from which the current knowledge of radiation-induced ocular injury were obtained, are from relatively high doses of photon radiation.
More recently, some ground-based studies have been conducted in rodent models to investigate low-dose space radiation-induced changes of ocular structure and function.
Studies show dose-dependent increases in apoptosis in the retina following space radiation exposure.
Data revealed that exposure to proton radiation-induced oxidative stress and apoptosis in the retina at a dose as low as 0.5Gy124.
Analysis of the microvasculature in the rat retina showed a time- and dose-dependent, progressive loss of endothelial cells and microvessel length over for two years after proton irradiation135.
Low doses of 16O ions also elicited apoptosis in the mouse retinal endothelial cells with the most robust changes observed after 0.1 Gy exposure compared to controls136.
Impact of Combined Exposure of Space Radiation and Simulated Microgravity on Ocular Structure and Function:
One of the main concerns for long-term deep manned space missions are health risks associated with combined exposure to microgravity environment and low-dose/low-dose-rate (LDR) radiation above levels normally found on earth due to GCRs.
It is an important contribution for risk assessment to determine whether the low dose radiation response is modulated by simulated microgravity.
The study design using a ground-based animal model to assess the biological effects of the spaceflight condition, combining space-like radiation exposure and microgravity is a more accurate model to simulate environmental stressors inherent to the spaceflight environment, providing a more actual risk assessment for astronauts.
To our knowledge, ground studies to examine the impact of the simulated space flight conditions and underlying mechanism(s) of potential interaction on retinal structure and functional damage are very limited.
In one study, mice were HLU for 7 days, then whole-body irradiated with protons at 0.5 Gy, followed by HLU for an additional 7 days.
The data showed SPE-like exposures of proton irradiation alone or combined with simulated microgravity has a significant impact on retinal endothelial cell survival71.
Additional and Future Considerations:
As noted earlier, factors and their interactions that contribute to detrimental ophthalmic changes to the spaceflight environment are not well-investigated.
More studies are needed to expose animals to microgravity simulation and low-dose space radiation simultaneously over at least 4 weeks which simulate the duration of the ISS mission.
This will allow the data to be extrapolated more accurately to estimate potential risks to astronauts in the space flight environment.
To simulate key aspects of space radiation exposures, further rodent study designs should consider exposing animals to both the charged particle composition of the radiation field and its low dose rate for ocular response measurements.
The observation periods for the study especially for degenerative tissues need to be extended for a longer time after radiation/unloading exposure to characterize the readaptation and chronic deficits.
One of the complications associated with determining the response of stress insults is the latency between exposure and the expression of injury (e.g., cell loss or dysfunction).
In order to obtain accurate data for the development and progression of the injury response, it is necessary to quantify changes over a long period.
The most profound physiological response and adaptation to the microgravity environment is the redistribution of fluid121.
The mechanisms by which fluid shift in the spacecraft environment that could affect ocular function were less studied.
Dedicated studies are needed to identify models to address this important question regarding the impact of the fluid shift on ocular structure, physiology, and visual function.
Further studies are also needed to specifically assess ocular perfusion pressure and ocular hemodynamics in appropriate animal models.
Electrophysiological assessment using electroretinogram (ERG) or other functional endpoints will be helpful to determine retina functional changes related to observed structural alteration.
Underlying cellular mechanisms of spaceflight environment, in facilitating ocular damage remain unclear.
Some lines of evidence suggest that one of the mechanisms involved in response to spaceflight, including changes in the gravity vector, is likely due to oxidative stress137,138.
Studies have shown that exposure to microgravity during spaceflights is associated with increased oxidative stress markers reflecting damage in lipid which results in lipid peroxidation in both humans and rodents139,140.
To expand our knowledge about the effects of spaceflight condition on the eyes and possible mechanisms associated with these changes, integrated omics profiling technologies such as genomics, proteomics and metabolomics are beneficial to determine sets of differentially expressed genes (DEGs), differentially expressed proteins, metabolomic/lipidomic signatures and the pathways that lead to pathological and possible degenerative changes.
Recently, RNA sequencing from a spaceflight study detected 600 DEGs in murine spaceflight retinas, which were enriched for genes related to visual perception, the phototransduction pathway, and numerous retina and photoreceptor phenotype categories141.
Delineating differential gene and protein expression and their relationship to overall pathophysiological and functional changes in the ocular tissue will provide a basis for the discovery and development of biomarkers and pathways for neurovascular changes in response to spaceflight condition.
Herein, using the “omics”-based molecular phenotyping approach for characterizing biosignatures associated with low-dose space radiation, simulated microgravity, and other space environmental stressors will help a deeper understanding of the underlying mechanisms responsible for the ocular structural and pathophysiological changes.
4. CENTRAL NERVOUS SYSTEM (CNS) RESPONSE
Importance of CNS Functionality to Mission Success:
Any deterioration in the ability of the astronauts to perceive or respond to changes in their situation could have disastrous consequences, as could changes in the mental health of the astronauts.
The ability of astronauts to successfully complete a deep space mission, such as the ones planned for Mars, will thus be highly dependent upon a fully functional CNS.
Not surprisingly, NASA has devoted considerable efforts to establishing the impact of social isolation, stress, sleep disturbances/loss and microgravity on various aspects of astronaut performance (cognition, sensorimotor response, social interaction, sleep) both during space flight and in rodent ground-based analogs.
In many cases there are well established procedures and interventions to detect and mitigate these stressor related issues.
However, there remains a high degree of uncertainty about the impact that exposure to space radiation will have on the cognitive and psychological capabilities of astronauts.
Microgravity Effects on the CNS:
Microgravity is also a major stressor on the CNS, inducing changes in the structure of the brain (rotation of the cerebral aqueduct, changes in ventricular volume, and narrowing of cerebrospinal fluid (CSF) spaces at the vertex142, and a cephalic fluid shift.
It also produces significant effects on the brain, particularly in cerebellar, sensorimotor, and vestibular brain regions (Reviewed in143).
Brain activity may also change in response to the need for increased processing required for postural stabilization, and integration of conflicting vestibular information in the microgravity environment144.
Despite all these changes, the evidence that prolonged microgravity leads to a permanent loss of cognitive function is sparse.
Astronauts report a “Space fog” for 1–2 days into a mission, but this typically resolves.
However, at the cellular level, simulated145 microgravity result in persistent changes in the mitochondrial function and lipid metabolism of human oligodendrocytes.
Oligodendrocytes are essential for providing metabolic support to neurons, rapidly transferring (through cytoplasmic “myelinic” channels and monocarboxylate transporters) short-carbon-chain energy metabolites like pyruvate and lactate to neurons146.
Such microgravity induced metabolic perturbations are likely to be deleterious to neuronal function in their own right, but will likely exacerbate changes in neuronal functionality with combined exposure to other spaceflight environmental hazards, such as radiation.
Space Radiation alters Neurophysiology and Neurocognitive Performance:
There is an ever-growing body of evidence from ground-based rodent studies that radiation exposure impairs performance in many cognitive processes, ranging from relatively fundamental processes to complex analogs/homologs of human cognitive tasks.
Even in the one study that demonstrates an apparent radiation-induced improvement in pattern separations skills147, mice demonstrated reduced associative memory formation ability, and the apparent improvement may be attributable to an increase in sparsely encoded hippocampal-dependent memory.
Thus, the overall consensus from ground-based rodent studies is that radiation exposure impacts performance in multiple cognitive tasks, utilizing multiple cognitive process governed by multiple brain regions.
Mechanistic studies have revealed multiple changes in neurophysiological processes and dendritic structure within most brain regions investigated148–159. Moreover, there may be a loss of connectivity between brain regions149.
Space Radiation Alone Impacts Cognitive Processes Deemed to Be of Operational Significance:
While it could be argued that the loss of the ability to perform a task like novel object recognition may not have any operational significance, the radiation-induced loss of performance in rodent versions of tests widely used to assess attention and cognitive flexibility in humans cannot be so easily discounted.
Astronauts are routinely screened during space flight for performance in a 10-test battery of cognitive tasks that NASA deemed necessary for mission success.
Seven of the tasks in the “fit-for-duty” performance battery160,161 assess some aspect of executive function.
Executive function, in lay terms, can be summarized as the “Triple A”: the ability to Assess, Adapt and Achieve.
More technically, executive functions are a set of higher order cognitive abilities that animals utilize to keep information ‘in mind’, attend to appropriate cues (e.g., nonverbal and verbal working memory stimuli), update information as contingencies change and invoke alternative, more appropriate responses to new situations.
The rodent version of the psychomotor vigilance test (rPVT) is virtually identical to the PVT test that is part of NASA’s “fit-for-duty” performance battery on the ISS160,161.
PVT performance is sensitive to fatigue, drug use, and age162,163, and exposure to mission-relevant (25 cGy) doses of protons results in deficits in accuracy, impulsivity and lapses in attention, all of which are indicative of deficits in sustained attention164.
Such lapses in attention account for 80% of flight accidents in the Navy and Marine Corps165.
A key process that allows humans to rapidly and efficiently adapt to different situations is task- or set-shifting.
An attentional set is formed when complex stimuli must be discriminated and classified as relevant or irrelevant to a particular task/situation.
Set-shifting can be simplistically thought of as the ability to relearn what the most important discriminating stimulus (for a particular endpoint) is in a changing environment.
Attentional set shifting (ATSET) thus enables subjects to rapidly adapt and respond to changes in the environment, and to perceive what is important for survival or completion of a task, skills that are required to deal with a sudden emergency.
The Wisconsin Card Sorting Test (WCST) has been widely used to assess task switching in humans166, with task switching deficits being increased in patients with Parkinson’s disease167,168 and autism169.
The intra-dimensional (IDS)/extra-dimensional (EDS) set shifting task is a modification of WSCT that assesses set-shifting abilities in rodents170.
Performance in the ATSET assay is impaired after exposure to ≤ 15 cGy of 1 GeV/n 56Fe155,171, 1 GeV/n 48Ti150,151, 600 MeV/n 28Si148 and protracted low dose rate neutrons172.
Astronauts on deep space missions will have to act more autonomously than on previous missions, especially when rapid responses to unexpected problems are required.
Creative problem solving skills will thus be of great importance to astronauts on a mission to Mars.
Recent studies have shown that low (≤18 cGy) doses of 600 MeV/n 28Si and 252Cf-generated neutrons impact creative problem solving in rats148,172.
Rather worryingly at the individual level, poor creative problem solving performance in the irradiated rats was not necessarily associated with poor ATSET performance, and vice versa148,172.
Previously we have shown that while space radiation exposure impairs both spatial memory and ATSET performance; however, when the relative performance of individual rats in each task was compared there was no correlation between space radiation-induced loss of performance in each task173.
These data suggest that risk assessments for radiation-induced neurocognitive impairment derived from a single cognitive domain may greatly underestimate the severity of the problem.
Exposing human colonization of the Moon and Mars as Space Fraud, Waste and Abuse
© 2025. All rights reserved. SpaceFraud.Tech